Next Launch
Total Students
2,609
Total Launches
683
Eggs Survived
418 61.2%
Rockets Survived
536 78.5%
Sept. 1, 2015
Measure the moon's size and distance during the next lunar eclipse
by Ethan Siegel
The moon represents perhaps the first great paradox of the night sky in all of human history. While its angular size is easy to measure with the unaided eye from any location on Earth, ranging from 29.38 arc-minutes1 (0.4897°) to 33.53 arc-minutes (0.5588°) as it orbits our world in an ellipse, that doesn't tell us its physical size. From its angular size alone, the moon could just as easily be close and small as it could be distant and enormous.
But we know a few other things, even relying only on naked-eye observations. We know its phases are caused by its geometric configuration with the sun and Earth. We know that the sun must be farther away (and hence, larger) than the moon from the phenomenon of solar eclipses, where the moon passes in front of the sun, blocking its disk as seen from Earth. And we know it undergoes lunar eclipses, where the sun's light is blocked from the moon by Earth.
Lunar eclipses provided the first evidence that Earth was round; the shape of the portion of the shadow that falls on the moon during its partial phase is an arc of a circle. In fact, once we measured the radius of Earth (first accomplished in the 3rd century B.C.E.), now known to be 6,371 km, all it takes is one assumption - that the physical size of Earth's shadow as it falls on the moon is approximately the physical size of Earth - and we can use lunar eclipses to measure both the size of and the distance to the moon!
Simply by knowing Earth's physical size and measuring the ratios of the angular size of its shadow and the angular size of the moon, we can determine the moon's physical size relative to Earth. During a lunar eclipse, Earth's shadow is about 3.5 times larger than the moon, with some slight variations dependent on the moon's point in its orbit. Simply divide Earth's radius by your measurement to figure out the moon's radius!
Even with this primitive method2, it's straightforward to get a measurement for the moon's radius that's accurate to within 15% of the actual value: 1,738 km. Now that you've determined its physical size and its angular size, geometry alone enables you to determine how far away it is from Earth. A lunar eclipse is coming up on September 28th, and this supermoon eclipse will last for hours. Use the partial phases to measure the size of and distance to the moon, and see how close you can get!
1 1 arc-minute = 1/60 of a degree. http://coolcosmos.ipac.caltech.edu/cosmic_classroom/cosmic_reference/angular.html
2 See http://resources.yesican-science.ca/eratosthenes/moon.html for a more detailed example of one way to measure the moon's radius.
This article was provided by the Jet Propulsion Laboratory, California Institute of Technology, under a contract with the National Aeronautics and Space Administration.
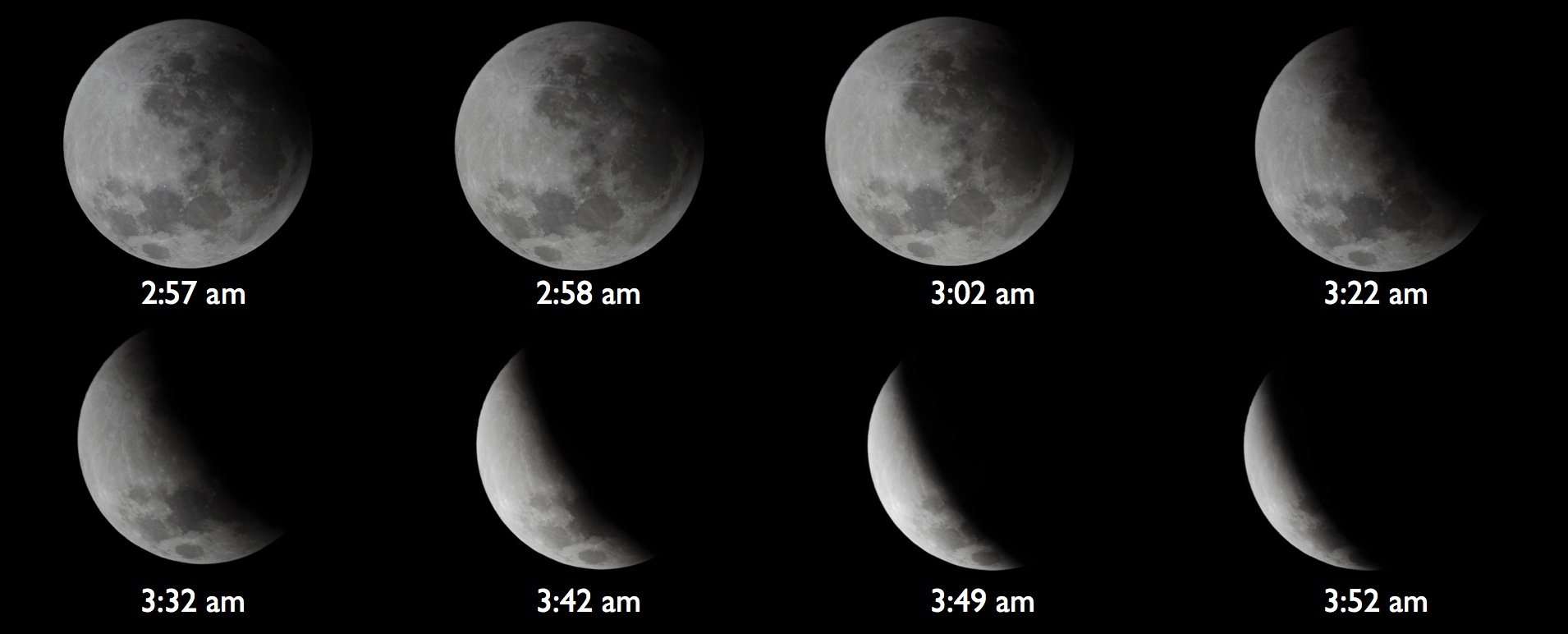
Oct. 1, 2015
How we know Mars has liquid water on its surface
by Ethan Siegel
Of all the planets in the solar system other than our own, Mars is the one place with the most Earth-like past. Geological features on the surface such as dried up riverbeds, sedimentary patterns, mineral spherules nicknamed "blueberries," and evidence of liquid-based erosion all tell the same story: that of a wet, watery past. But although we've found plenty of evidence for molecular water on Mars in the solid (ice) and gaseous (vapor) states, including in icecaps, clouds and subsurface ices exposed (and sublimated) by digging, that in no way meant there'd be water in its liquid phase today.
Sure, water flowed on the surface of Mars during the first billion years of the solar system, perhaps producing an ocean a mile deep, though the ocean presence is still much debated. Given that life on Earth took hold well within that time, it's conceivable that Mars was once a rich, living planet as well. But unlike Earth, Mars is small: small enough that its interior cooled and lost its protective magnetic field, enabling the sun's solar wind to strip its atmosphere away. Without a significant atmosphere, the liquid phase of water became a virtual impossibility, and Mars became the arid world we know it to be today.
But certain ions - potassium, calcium, sodium, magnesium, chloride and fluoride, among others - get left behind when the liquid water disappears, leaving a "salt" residue of mineral salts (that may include table salt, sodium chloride) on the surface. While pure liquid water may not persist at standard Martian pressures and temperatures, extremely salty, briny water can indeed stay in a liquid state for extended periods under the conditions on the Red Planet. It's more of a "sandy crust" like you'd experience on the shore when the tide goes out than the flowing waters we're used to in rivers on Earth, but it means that under the right temperature conditions, liquid water does exist on Mars today, at least in small amounts.
The measured presence and concentration of these salts, found in the dark streaks that come and go on steep crater walls, combined with our knowledge of how water behaves under certain physical and chemical conditions and the observations of changing features on the Martian surface supports the idea that this is the action of liquid water. Short of taking a sample and analyzing it in situ on Mars, this is the best current evidence we have for liquid water on our red neighbor. Next up? Finding out if there are any single-celled organisms hardy enough to survive and thrive under those conditions, possibly even native to Mars itself!
This article is provided by NASA Space Place.
With articles, activities, crafts, games, and lesson plans, NASA Space Place encourages everyone to get excited about science and technology. Visit spaceplace.nasa.gov to explore space and Earth science!
This article was provided by the Jet Propulsion Laboratory, California Institute of Technology, under a contract with the National Aeronautics and Space Administration.
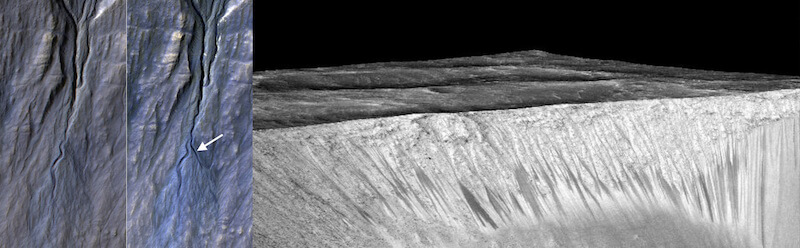
Nov. 1, 2015
Our Solar System Is Almost Normal, But Not Quite
by Ethan Siegel
It was just over 20 years ago that the very first exoplanet was found and confirmed to be orbiting a star not so different from our own sun. Fast forward to the present day, and the stellar wobble method, wherein the gravitational tug of a planet perturbs a star's motion, has been surpassed in success by the transit method, wherein a planet transits across the disk of its parent star, blocking a portion of its light in a periodic fashion. Thanks to these methods and NASA's Kepler spacecraft, we've identified many thousands of candidate planets, with nearly 2,000 of them having been confirmed, and their masses and densities measured.
The gas giants found in our solar system actually turn out to be remarkably typical: Jupiter-mass planets are very common, with less-massive and more-massive giants both extremely common. Saturn-the least dense world in our solar system-is actually of a fairly typical density for a gas giant world. It turns out that there are many planets out there with Saturn's density or less. The rocky worlds are a little harder to quantify, because our methods and missions are much better at finding higher-mass planets than low-mass ones. Nevertheless, the lowest mass planets found are comparable to Earth and Venus, and range from just as dense to slightly less dense. We also find that we fall right into the middle of the "bell curve" for how old planetary systems are: we're definitely typical in that regard.
But there are a few big surprises, which is to say there are three major ways our solar system is an outlier among the planets we've observed:
- All our solar system's planets are significantly farther out than the average distance for exoplanets around their stars. More than half of the planets we've discovered are closer to their star than Mercury is to ours, which might be a selection effect (closer planets are easier to find), but it might indicate a way our star is unusual: being devoid of very close-in planets.
- All eight of our solar system's planets' orbits are highly circular, with even the eccentric Mars and Mercury only having a few percent deviation from a perfect circle. But most exoplanets have significant eccentricities, which could indicate something unusual about us.
- And finally, one of the most common classes of exoplanet-a super-Earth or mini-Neptune, with 1.5-to-10 times the mass of Earth-is completely missing from our solar system.
Until we develop the technology to probe for lower-mass planets at even greater distances around other star systems, we won't truly know for certain how unusual we really are!
With articles, activities, crafts, games, and lesson plans, NASA Space Place encourages everyone to get excited about science and technology. Visit spaceplace.nasa.gov to explore space and Earth science!
This article was provided by the Jet Propulsion Laboratory, California Institute of Technology, under a contract with the National Aeronautics and Space Administration.
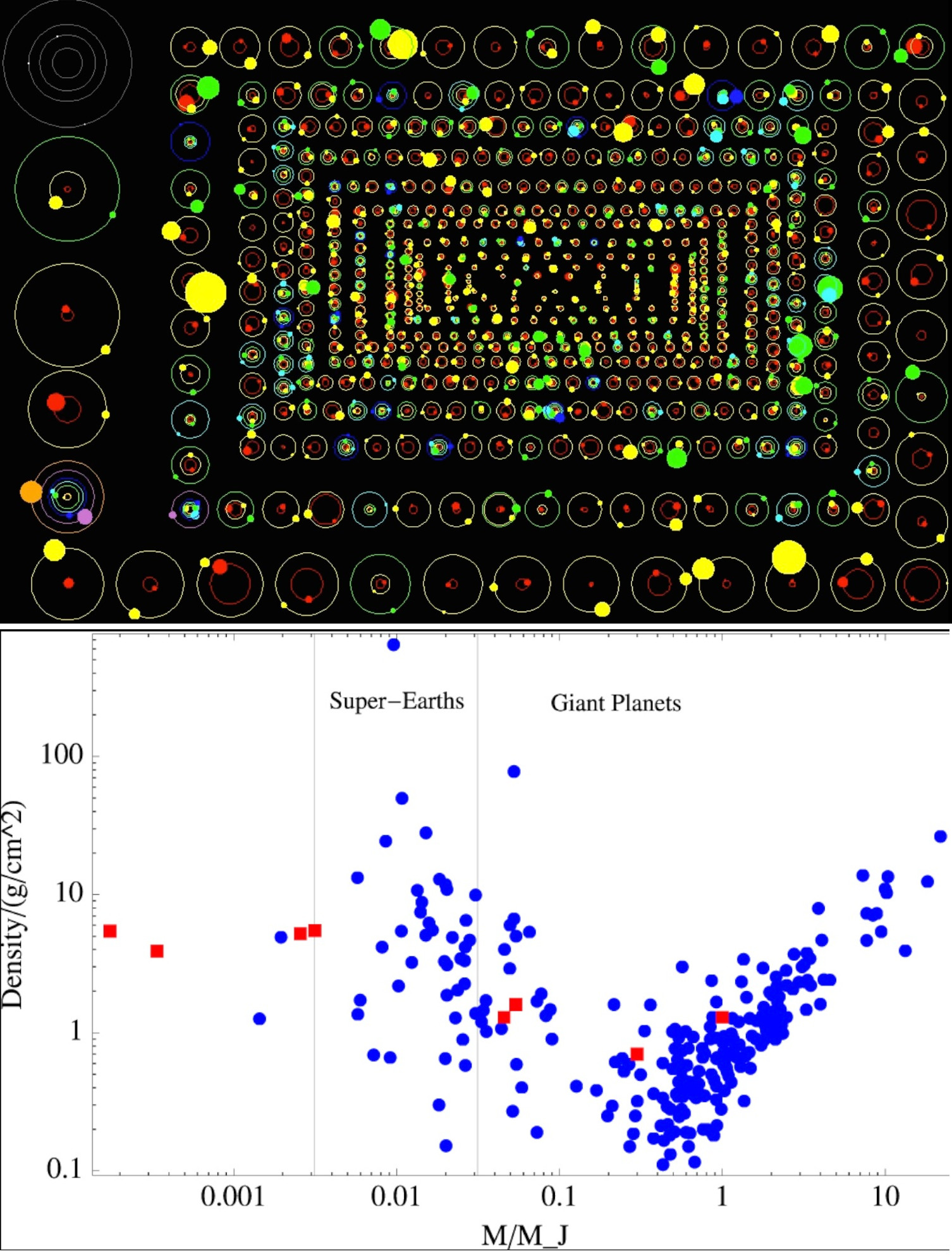
Dec. 1, 2015
How will we finally image the event horizon of a black hole?
by Ethan Siegel
One hundred years ago, Albert Einstein first put forth his theory of General Relativity, which laid out the relationship between spacetime and the matter and energy present within it. While it successfully recovered Newtonian gravity and predicted the additional precession of Mercury's orbit, the only exact solution that Einstein himself discovered was the trivial one: that for completely empty space. Less than two months after releasing his theory, however, the German scientist Karl Schwarzschild provided a true exact solution, that of a massive, infinitely dense object, a black hole.
One of the curious things that popped out of Schwarzschild's solution was the existence of an event horizon, or a region of space that was so severely curved that nothing, not even light, could escape from it. The size of this event horizon would be directly proportional to the mass of the black hole. A black hole the mass of Earth would have an event horizon less than a centimeter in radius; a black hole the mass of the sun would have an event horizon just a few kilometers in radius; and a supermassive black hole would have an event horizon the size of a planetary orbit.
Our galaxy has since been discovered to house a black hole about four million solar masses in size, with an event horizon about 23.6 million kilometers across, or about 40 percent the size of Mercury's orbit around the sun. At a distance of 26,000 light years, it's the largest event horizon in angular size visible from Earth, but at just 19 micro-arc-seconds, it would take a telescope the size of Earth to resolve it - a practical impossibility.
But all hope isn't lost! If instead of a single telescope, we built an array of telescopes located all over Earth, we could simultaneously image the galactic center, and use the technique of VLBI (very long-baseline interferometry) to resolve the black hole's event horizon. The array would only have the light-gathering power of the individual telescopes, meaning the black hole (in the radio) will appear very faint, but they can obtain the resolution of a telescope that's the distance between the farthest telescopes in the array! The planned Event Horizon Telescope, spanning four different continents (including Antarctica), should be able to resolve under 10 micro-arc-seconds, imaging a black hole directly for the first time and answering the question of whether or not they truly contain an event horizon. What began as a mere mathematical solution is now just a few years away from being observed and known for certain!
Note: This month's article describes a project that is not related to NASA and does not suggest any relationship or endorsement. Its coverage is for general interest and educational purposes.
This article is provided by NASA Space Place. With articles, activities, crafts, games, and lesson plans, NASA Space Place encourages everyone to get excited about science and technology. Visit spaceplace.nasa.gov to explore space and Earth science!
This article was provided by the Jet Propulsion Laboratory, California Institute of Technology, under a contract with the National Aeronautics and Space Administration.
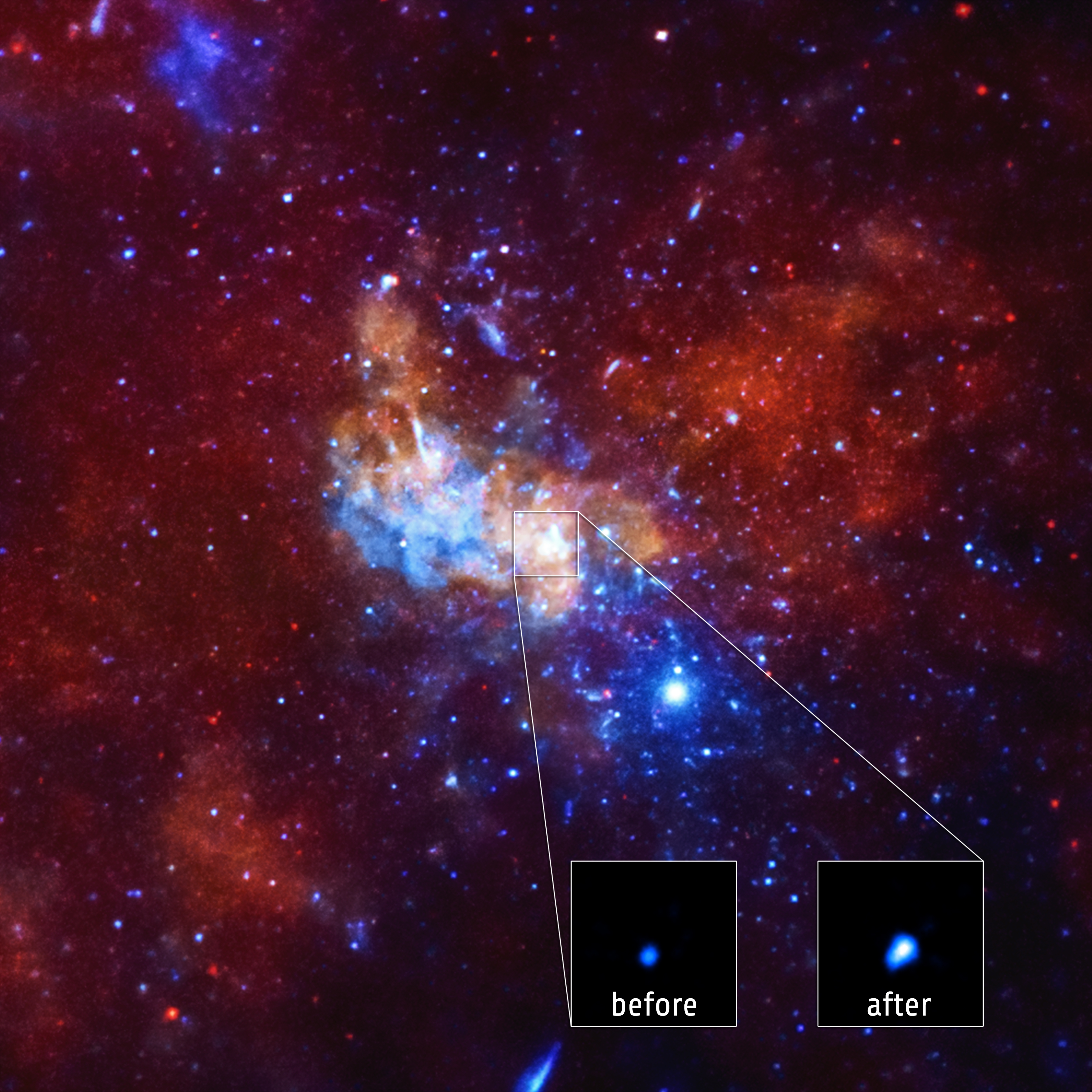